Does a theory of everything exist?
Some scientists question if the guiding lights of theoretical physics have gone out, and all wonder where the field will go next
Charlie Wood • August 13, 2018
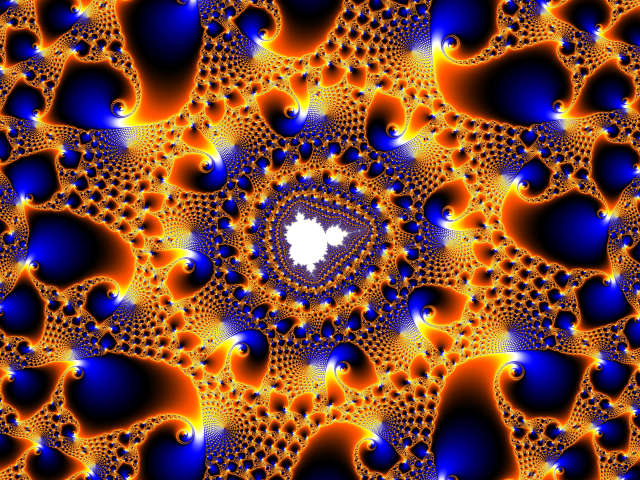
No matter how far you zoom into a fractal (a shape that continually repeats its geometry at smaller and smaller scales), new details keep coming into focus. Could reality have a similar structure? [Image courtesy of Robert Sontheimer | CC BY 3.0]
Flying is a miracle. The vibrating floor tickles the soles of my feet, perhaps from the jet engines hurling us forward, or maybe from the flexing of the wings as wind currents buffet them. Air hisses as it circulates, maintaining just the right pressure to keep my ears (relatively) comfortable. Despite the smooth ride, the orange glow of the seatbelt sign tells me that things are about to get bumpy, meteorological information that entered the plane via radio waves, before the flow of electricity relayed it to us passengers.
And yet this engineering marvel rests on humdrum physics. Nothing here would surprise a 19th century scientist, except perhaps that traveling from New York to Tokyo takes just twelve hours. It wasn’t until the 20th century that physicists drilled down into more fundamental levels beyond the familiar world of winds, metals, currents and waves.
The work of those researchers established three essentially independent realms of reality, each hidden inside the last. Materials are made up of elements like gold and oxygen, different species of atoms. Peer inside an atom, and you’ll find a cloud of electrons buzzing about a ball of protons and neutrons. Zoom in even further, and each proton and neutron resolves into a trio of particles called quarks — bouncing around at nearly the speed of light but chained together by an unbreakable bond. The description of these particles, along with other rarer species and their interactions, evolved into our most complete rulebook for the universe yet: a single equation you can fit onto a coffee mug.
“From the point of view of understanding nature, the Standard Model is just a fantastic construction,” says Adam Falkowski, a particle physicist working at the Laboratoire de Physique Théorique in France. “A perfect example of human achievement.”
But confirmation of the final particle of that picture came in 2012, and attempts to improve upon that triumph have triggered a crisis. For decades, physicists have known what ought to come next. A common desire that the universe be mathematically beautiful hinted that a new class of particles could add a sense of balance — called supersymmetry — to the Standard Model, explaining some eye-popping coincidences and paving the way for more ambitious models.
Unfortunately, nature had other plans. Despite nearly ten years of hunting, the Large Hadron Collider at CERN has not detected the most likely set of supersymmetric particles, an experimental result with deep theoretical consequences. A generation of physicists devoted their careers to the notion of supersymmetry, and while the mathematical tools they developed will surely find use elsewhere, many suggest the field’s guiding philosophy needs an overhaul. Simply put, while some physicists thought nature’s bottom level was nearly in sight, many now find themselves staring into opaque depths — some questioning whether there is a bottom to be found at all.
“I used to love to say that I’m a scientist because I want to understand the ultimate nature of reality,” says Marcelo Gleiser, a theoretical physicist at Dartmouth College, “but nowadays I’m like, what the heck does that even mean?”
Hungry for Standard Model errors that might point the way toward new physics, experimentalists are going to ever greater lengths. In February at Illinois’s Fermilab, researchers started shooting muons (a heavy cousin of the electron) around a magnetic ring in the hopes of determining whether a nearly twenty-year-old measurement is a fluke or a sign.
The particles should turn at a certain rate as they move — a rate the Standard Model can predict to ten decimal places. But a 2001 measurement made at Long Island’s Brookhaven National Laboratory found something unexpected. Although it seems unbelievable, muons (and all particles) actually swim through what Fermilab physicist Chris Polly calls “a sea” of short-lived particles that constantly flash into and out of existence, tweaking their paths. The Brookhaven experiment measured a detour from that path in a way that can’t be explained by the Standard Model. Today, some hope that the deviation could represent unknown particles in the sea’s froth jostling the muons.
The size of the anomaly? Less than three ten-thousandths of a percent discrepancy with the Standard Model prediction. “That’s the part we’re interested in,” Polly says, “because that’s the part that’s driven by all the other particles in the universe [the muon] interacts with.” Brookhaven’s result wasn’t precise enough to distinguish between new particles and statistical bad luck, but Fermilab’s upgraded equipment should be able to tell the difference.
Why go to all this trouble when the Standard Model works so well? Because everyone knows it can’t be the whole story. Two types of reasons push physicists to reach for more fundamental theories. Some scientists label them “experimental facts” and “aesthetics,” but you could just as easily call them the “bucket list” and the “wish list.”
The bucket list is all problems that must be solved because something weird is going on. Galactic spin, for example, reveals the tug of unseen mass, indicating either a new type of matter or a failure of our theory of gravity. What’s more, either an unknown force is pushing the universe to expand faster and faster, or our interpretation of cosmological data is deeply flawed. Any complete theory must account for these observations of so-called dark matter and dark energy.
“They tell us there must be something,” Falkowski says. “Unfortunately, they don’t tell us where.”
Paradoxically, what often told theorists where a theory was going wrong in the past was the wish list — the ugly aspects of the model they’d like to explain more elegantly. The colliders of the ‘40s and ‘50s, for instance, produced a mess of new particles that researchers nicknamed “the particle zoo.” Most scientists doubted nature’s so-called building blocks could be so numerous, and sure enough many of those particles turned out to be made up of quarks.
Mathematically-driven simplifications continued through the ‘80s, says Gian Giudice, head of CERN’s theory department. “Because of the tremendous success of the Standard Model, most came to the realization that following the same logic of symmetry, you could make progress.” He recounts a heady era where the emergence of supersymmetry and an exotic idea called string theory made it seem like math could win the race to the bottom. “There was a conception that this game should continue,” he says.
But in their race down the modern wish list, theorists seem to be hitting a wall. One rationale for supersymmetry was the suspiciously light mass of the Higgs boson, the particle that interacts with others to give them mass. Some calculations predicted that interactions with the bizarre sea of temporary particles (like in the Fermilab muon experiment) should make the Higgs huge, but when measurement confirmed it to be impossibly light, theorists proposed the existence of supersymmetric particles to balance it out. In hindsight, some now question the philosophy behind that assumption.
“I’m very glad I did not spend my career working on supersymmetry,” Gleiser says. “I gradually realized that this notion that there’s this perfect symmetry behind everything is almost like a religion.”
Physicists also wish to understand why certain particles have certain masses, and whether the various forces are actually variations of one primordial force. Still, they recognize that solving these problems is not strictly necessary. “There are some questions that don’t have good answers,” admits Polly, offering solar eclipses as a common counterexample. The moon just happens to have the right size and orbit to perfectly block the sun from the Earth’s surface, but nothing made it form that way. Sometimes a coincidence is just a coincidence.
As certain aesthetic qualities like symmetry may be falling out of vogue, this intellectual culling could be just what physics needs to stimulate fresh ideas. “In science, the best moments are the moments of confusion,” says Giudice, who spells crisis with a “k” to include the ancient Greek connotation of “turning point.”
He likens our current approach to a biologist trying to establish a complete theory of zebras by measuring stripes and counting individuals. She may learn a lot about zebra appearance and population number, but how they came to be that way would seem random and improbable, like the Higgs mass or the moon’s size. Only after developing theories of lions, predation and evolution would she have a hope of answering “why” questions about falling populations and stripe patterns. Similarly, Giudice sees the current “krisis” as an opportunity for a revolution resembling Darwin’s theory in its ability to link unrelated domains.
Until now, physics has moved step-by-step into ever smaller, but separate realms. First atoms, then sub-atomic particles, then quarks. This approach worked because the levels scarcely interact: You can do chemistry or build an airplane without even knowing about quarks. But that separation could be just a fluke of the scales accessible via particle accelerators, and we may have gotten as far as we can with the one-step-at-a-time approach.
Outstanding problems such as the Higgs mass and the nature of dark energy seem to sprawl across multiple levels, making them hard to crack with familiar tools. “When you look at it at the quantum level,” Giudice says, “you realize that [these phenomena] actually embed information about many different scales.”
So how deep could this rabbit hole go? Maybe science fiction novelist Douglas Adams got it right when he wrote: “There is a theory which states that if ever anyone discovers exactly what the Universe is for and why it is here, it will instantly disappear and be replaced by something even more bizarre and inexplicable. There is another theory which states that this has already happened.”
But ask five physicists whether there’s one true nature of reality, and you’ll get five polite refusals to engage in speculation. “If we stay at the philosophical level,” says Patrick Koppenburg, a particle physicist hunting for whiffs of new physics at the LHC with the Dutch National Institute for Subatomic Physics, “it’s possible that there is nothing, or that it is at such a scale that it is totally out of reach for humanity, which effectively is the same thing.”
Push harder, and you’ll get dozens of responses, most of which can be squeezed into one of two frameworks. Either there is an ultimate level (although it may be inaccessible), or the levels keep going indefinitely (more than one person I talked to for this essay labeled the idea “turtles all the way down”). Regardless, no one’s worried about job security.
“I don’t think that we, as humans, will ever be satisfied,” Polly says. “Every question that we find an answer for ends up generating two more questions.”
Even if theorists did hit on the ultimate theory of everything, they still couldn’t be certain nature wasn’t hiding another trick up her sleeve. “Everything that we know about depends on instruments,” Gleiser says. Theory springs from observation, and all observations have their limits. “We are always, always partially blind.”
We humans may struggle to discern the architecture of reality — be it particle nesting dolls, strings, turtles, or something else altogether — but nature knows what game she is playing. She does not jump from chess to Monopoly (although apparently, she does love dice). “We have discovered that nature does not work randomly,” says Giudice. “There is an order in nature, which is expressed by physical laws. It seems almost unbelievable that this should not persist at deeper levels.”
I, for one, am glad nature follows one rulebook, even if it’s hard to read and full of finely printed, telescoping footnotes. I’m reasonably sure that my plane will land on schedule, and it’s a relief to know that if there’s a discrepancy, it will arise from thoroughly logical (although perhaps unknowable) reasons.
Editor’s note: A previous version of this article mistakenly stated Adam Falkowski’s professional affiliation. It is the Laboratoire de Physique Théorique in Orsay, France.