Eavesdropping on the brain
Scientists use neural prosthetics to supplement brain functions
Knvul Sheikh • January 10, 2016
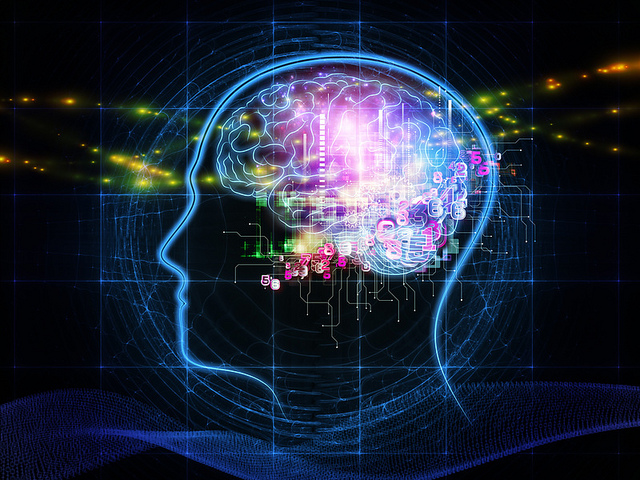
Scientists can “listen” to brain activity and use neural prosthetics to improve a variety of functions [Image credit: Flickr user A Health Blog]
What if a brain implant could enhance your strength, vision or hearing? Or what if a small memory chip, wired directly into your brain, could give you perfect recall? And what if a new type of medical device implanted on a nerve could read your vital signs and analyze them for disease progression, perhaps even preventing a seizure or stroke before it happened?
Over the past few decades, we as a society have become comfortable with medical devices being implanted in various parts of our bodies, such as pacemakers for the heart, artificial hips and prosthetic limbs. But now this idea of prosthetic supplementation has reached the brain. Advances in both neuroscience and technology have allowed scientists from around the world to start understanding the brain well enough to have direct conversations with it. And researchers hope to use this technology to help people overcome all sorts of impairments.
Because nerves go everywhere in the body, researchers can potentially use these devices for more than just neural deficits. Neural prostheses could help improve any bodily function, ranging from hormone control to enhancing the immune system to lowering blood pressure.
“Neural prostheses can become a tool to interact with the nervous system to fix a wide variety of medical problems,” says Cindy Chestek, a biomedical engineer at the University of Michigan who studies how brain signals can be re-routed to improve prostheses for paralyzed people.
But before neural implants can bypass damaged brain tissue, scientists need to have a map of the brain as well as a phrase book to become fluent in brain “language.” Until a few years ago, this brain map was very fuzzy — kind of like the world maps early explorers used.
Now researchers have added to their brain phrase book and made a clearer map of brain activity by inserting electrodes in the brain. The electrodes pick up electrical pulses, which carry the communication between the brain’s cells, or neurons. And variations in the strength of these signals, that is, the connections between neurons, allow the brain to analyze patterns, such as a familiar face or predict how far your arm needs to reach out to press the snooze button on your alarm in the morning.
By eavesdropping on the brain’s electrical activity, scientists can decode how signals are stored as memories, for example. An implant placed directly on the brain’s hippocampus, where short-term memory is converted into long-term memory, could simulate calculations the hippocampus makes in determining where each memory is stored. Such prostheses could then enhance subjects’ recall of a particular task or event.
Because the who, what, where and why of a memory can involve motion and sensory input, as well as other types of information, researchers like Dong Song, an associate professor at the University of Southern California in Los Angeles, have to first piece together bits of information from each neuron to make up the whole picture that forms a single memory.
“[Memory] is very complex. It’s non-linear and very dynamic, meaning it depends on a lot of different factors,” says Song. He and his collaborators have already progressed from testing how their model simulates all this information from hippocampal implants in animal brains to a prototype for human clinical trials.
“The next step, which hasn’t been done yet, is to stimulate the downstream signal from the hippocampus,” says Song. He explains that this would help close the loop — reading input signals for a particular memory, analyzing the information, and then storing the output.
Once hippocampal implants can handle both input and output information, Song hopes that they will be helpful for epileptic patients who suffer from bouts of amnesia due to their seizures.
“It is a brute force method of increasing the standard of living of people suffering from memory loss or neurodegenerative disease,” says Song’s collaborator Clayton Bingham, who is also a researcher testing hippocampal implants at the University of Southern California.
Other implants, such as those designed in Chestek’s lab to improve traditional prosthetic limbs, also use computer models. They decode neural signals that control movement. Chestek says these tiny implants, which are the size of a pinky fingernail, have enabled paralyzed patients to do everyday tasks we take for granted, like feeding themselves and brushing their teeth.
“Right now we’re trying to get more degrees of freedom,” says Chestek. “Most people using traditional systems can only do open-close movements. Whereas for the neural control systems, people can imagine four or five different movements [of fingertips] and control them.”
Similar principles for translating information can be applied to neural prostheses that write into the brain instead of reading from it. Two years ago, the FDA approved a clinical trial of retinal prostheses that take images from a small camera mounted on one’s glasses and turn those images into electrical pulses, which are then delivered on a chip implanted on the back of the eye. James Weiland, a biomedical engineer at the University of Southern California whose team is leading these retinal prostheses trials, says they stimulate the retinal nerve like the eye normally does, thus potentially restoring sight to the blind.
But how close are we to having such wondrous devices easily available for everyone? To begin with, scientists and engineers need to figure out how to package these devices into smaller, more efficient systems.
“There’s a trade-off between how much power you have available to run the device versus how large your device can be,” says Weiland.
Another concern is that the implant must be nontoxic and biocompatible so as not to provoke an immune system reaction. Currently, all electronics implanted in the body — even pacemakers — have to be placed inside a titanium or ceramic casing to increase how long they last inside the body.
“The body is a very harsh environment,” says Chestek. “It’s very warm, it’s very salty, and it actively attacks anything that is not it.”
Electrodes that poke out of the titanium encasing must also be made of materials that are flexible yet stiff enough to insert in the brain. Chestek’s lab, for example, is developing carbon fiber electrodes for this purpose. And while each electrode is even smaller than the size of a human hair, it is still not small enough. Any increase in the number of electrodes, which would otherwise increase accuracy of the signals recorded, would make the device too big to be implantable.
These obstacles may seem daunting, but researchers just need to figure out ways to miniaturize current technology, says Chestek. It’s similar to how cell phones were a few years ago.
Once researchers learn how to overcome these hurdles, neural prosthetics could become more widely used to treat diseases such as depression, Alzheimer’s and epilepsy that have traditionally been difficult to treat with drugs. In fact, scientists are already expanding their knowledge of how the nervous system works to manipulate other systems, such as the inflammatory response in rheumatoid arthritis or Crohn’s disease.
And Chad Bouton, managing director of the Center for Bioelectronic Medicine at the Feinstein Institute in Manhasset, New York, says that neural prosthetics could even eliminate the need for drugs in some disorders. “If we learn how to tap into the human nervous system and in essence reprogram it,” Bouton says, “it is very possible that in the future this approach could eclipse the use of pharmacologics.”
Eventually, neural prosthetics could open up possibilities that we can scarcely imagine, says Bouton. “The sky is the limit.”