Plastics don’t break — they waltz
Chemists are reimagining how polymer chains respond to physical stress, inviting innovative approaches to medicine, tech and more
Gayoung Lee • December 6, 2023
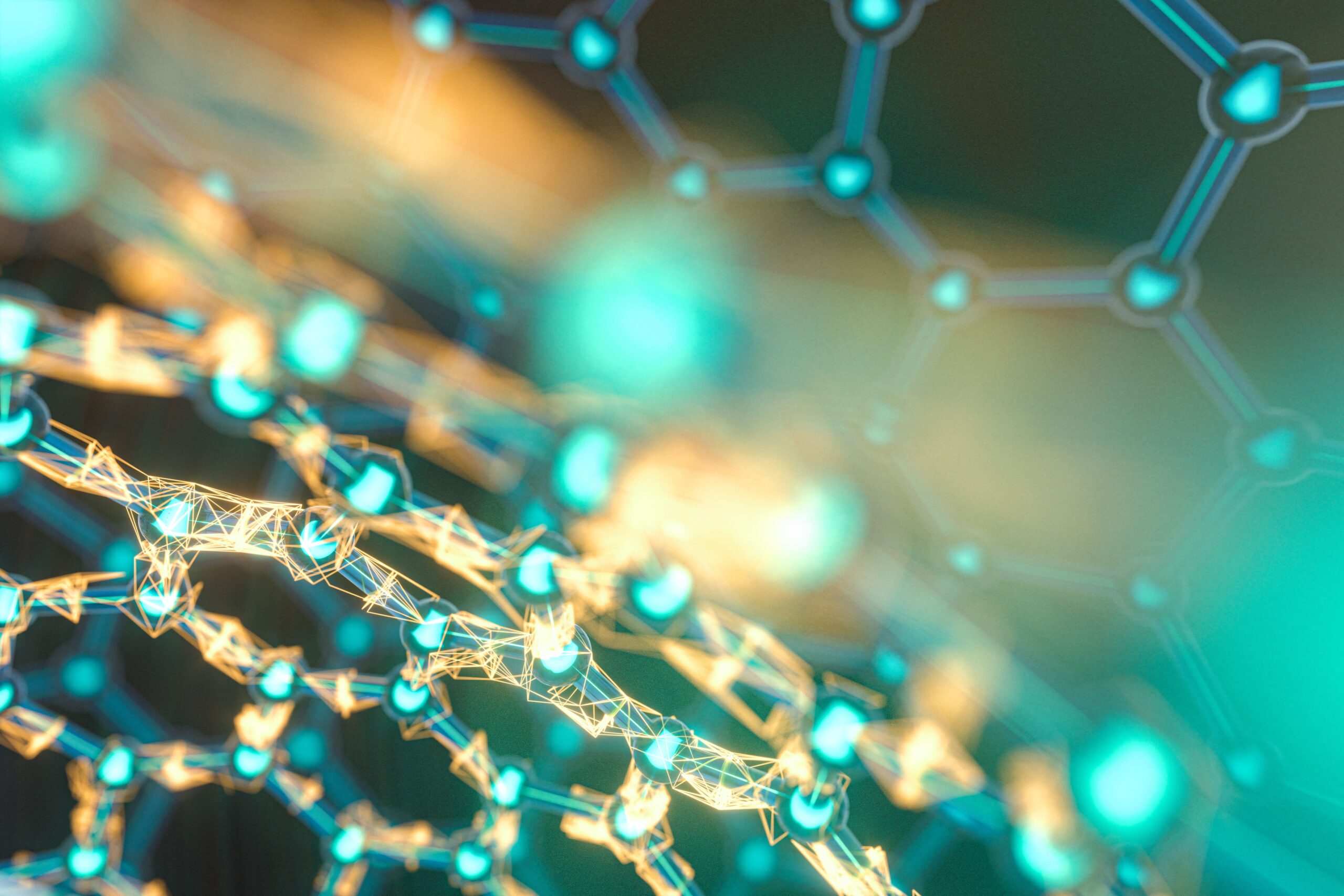
Networks of molecules form polymer structures responsible for things such as hardness or elasticity in plastics, clothes and even airplanes [Credit: Vink Fan | CC BY 2.0]
The next time you snap a rubber band, tear a sweater or chip a nail, beware: you might have just kicked off a molecular dance party.
Rubber, wool and fingernails are all made of polymers, which are hundreds to thousands of identical chemically-bonded molecule chains. The intricate geometry of these polymer chains creates an optimal combination of strength and flexibility in all sorts of manufactured products, from tires and heat-resistant airplanes to the circuit boards in cellphones.
But even polymer chains can break under enough pressure. What that means functionally will vary depending on the type of polymers, but often this means the deterioration of a certain quality we expect from a polymer-based product. For instance, heat-resistant airplanes may lose their ability to withstand high temperatures, or the circuit boards in cellphones might have trouble conducting electricity. So, polymer chains’ ability to stay intact has a lot of influence on whether everyday products work the way we want them to.
That’s why for researchers and engineers, understanding polymer behavior on the nanoscopic level plays an important role in designing stronger polymers, as well as discarding and recycling them more efficiently. Down the road, material scientists believe polymers could be used in the development of new materials that deliver drugs with unprecedented precision or even repair themselves when broken.
Now, researchers at the University of Liverpool are proposing a new way to think about what happens when polymers break, opening up possibilities for better materials. They suggest that the molecules in these long molecular chains do something unexpected when put under stress: they shift and “dance.”
The researchers laid out this surprising concept in a recent paper in Nature Chemistry, proposing a dynamic alternative to the long-held assumption that polymers simply break straight down the middle when overstretched.
The old idea “just made sense in terms of math and physics — when you pull on a chain, it breaks from the center, where the most force is,” explains Charles Diesendruck, a chemist at Technion, the Israeli Institute of Technology, who was not involved in the study.
But how polymers behaved in actual experiments performed by chemists like Diesendruck betrayed what would be predicted by physics. Instead, under stress, polymers would always break off at points slightly removed from the center.
What authors Robert O’Neill and Roman Boulatov propose helps explain what Diesendruck has observed under the microscope in the nanoseconds after a polymer chain breaks. Reacting 100 million times faster than the blink of an eye, a polymer chain completes a shift that is tantamount to a nanoscopic waltz sequence.
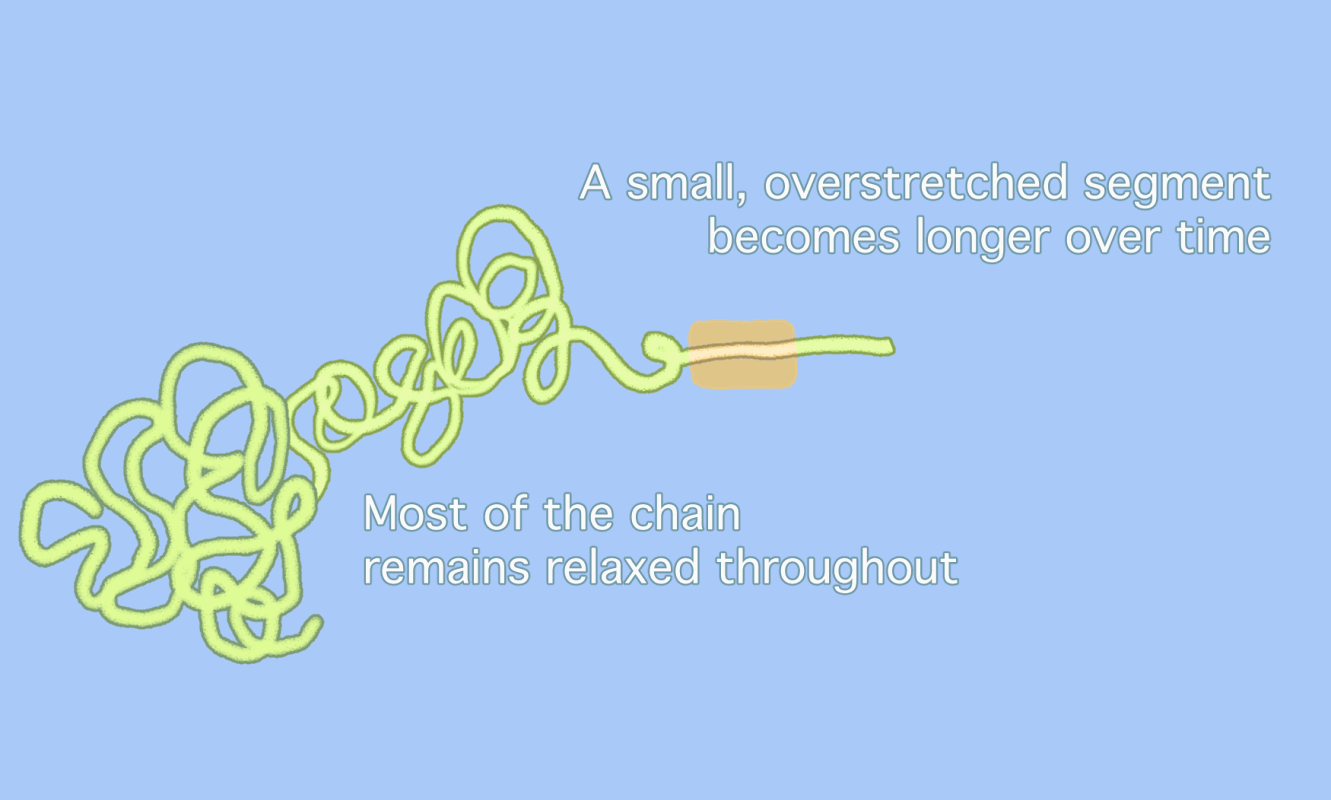
Chemists have long assumed that polymer chains, when subjected to enough pressure, simply snap in the middle, as the animation on the top shows. But a new idea (bottom) suggests that overstretched segments of polymer chains only unravel in part, with a small, overstretched segment moving back and forth until the segment itself snaps at a point slightly removed from the center. This sequence makes it look as if parts of the polymer “dance” back and forth along the chain [Credit: Gayoung Lee]
To come up with their new model for what happens at the molecular level when polymers are stressed, O’Neill and Boulatov watched how nine polystyrenes (popularly known as styrofoam) in a liquid solution reacted as they were struck by ultrasound. From their observations, they derived a mathematical representation of how polymer chains “flow” in response to physical impact.
A polymer chain under stress “never is a complete, straight rod,” Boulatov says. “It’s more like there is a blob with a rod-like piece that moves around and grows in size, and the chain breaks because it stretches so much, but the break is not located exactly at the center.”
Pasta analogies are one way to think about polymer geometry, according to Matthew Golder, a chemist at the University of Washington who was not involved in the study. Most polymer chains resemble the blob-like shape of “cooked spaghetti,” Golder wrote in an email. If the previous idea assumed that one huge strand of spaghetti was overstretched until it broke, the new idea suggests that most of that spaghetti remains “relaxed” in a blob. Only a small section of that spaghetti strand moves back and forth between the blob and the end of the strand. Because most of the polymer blob has not been “unwrapped” when the break happens, the break will always take place away from the exact center of the longer chain.
How polymers behave under stress is a key consideration in the assembly — and disassembly — of many plastics and other polymer-based materials. For example, mechanical force could become an alternative to disposal processes for certain types of plastic that currently rely on chemical solvents to dissolve polymer bonds.
“If we are able to define what happens at that level,” Diesendruck says, “we might be able to prevent material failure at the level we can see.”
Theoretically, a polymer used on an iPhone screen could be designed to “activate” only when the screen breaks — that is, when mechanical stress is applied — and the polymers then would reattach themselves, fixing the screen without any outside assistance, according to Diesendruck.
New polymers designed with detailed knowledge of reactions at the molecular level could have potential medical uses, too, Boulatov adds. For example, they could be constructed to carry small drug molecules that are immediately released when the chain breaks.
“Polymers are interesting because they are such stable structures at room temperature, but so easy to break — it’s so easy to snap a rubber band, for example,” Boulatov says. “The more we understand polymer kinetics, the more we can expand the limits of polymers to work exactly the way we want and need it to be.”