Hacking photosynthesis to feed the future
Pesticides, fertilizers, and machines aren’t enough to meet the burgeoning demand for food crops but fast-growing GM plants could pick up the slack
Tara Santora • July 20, 2019
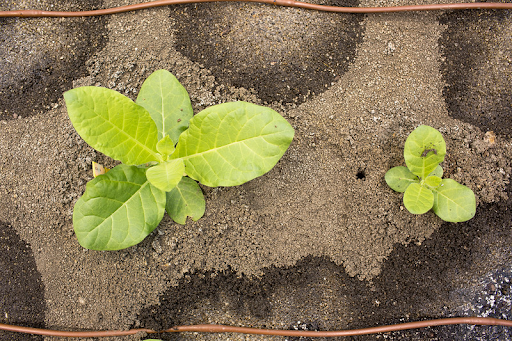
An unmodified tobacco plant (right) grows beside one that has been genetically engineered to circumvent an energy-draining inefficiency in photosynthesis. [Credit: Claire Benjamin/RIPE Project, with permission]
Paul South tries to curb his excitement as he walks through the greenhouse at the University of Illinois, but the evidence before him makes that difficult. His genetically engineered tobacco plants are — no doubt about it — dominating their unmodified cousins. Seeing the two next to each other is like watching a teenage boy on a growth spurt puff out his chest next to his pipsqueak sibling.
“Something big is probably happening here,” South says. And it’s not just the size of the plant.
By altering a gene to make photosynthesis more efficient, South and his team have increased the mass of tobacco plants by over 40 percent in the field, according to a recently published study in the journal Science. A molecular biologist at the U.S. Department of Agriculture, South hopes this proof-of-concept work will translate to similar results in crops like soybeans, rice, and wheat.
South says crops will need these sort of gene edits to meet the increasing demands of a world hungry for biofuel, meat and dairy. Farmers will need to double crop production by 2050 to meet global demands, but agricultural improvements are not on track to fix this problem, according to a 2013 analysis.
Crop yields exploded in the 1960s, thanks to new farming techniques such as breeding high-yield varieties of staple crops and using better pesticides, fertilizers, and mechanical harvesters. But these improvements are now increasing crop productivity by less than two percent per year, which is not enough to meet future food demand, according to South. Making photosynthesis more efficient, he adds, is one promising area that hasn’t yet been fully realized.
During photosynthesis, a plant enzyme called rubisco pulls carbon dioxide out of the air, which plants need for growth. But once every four or five times, rubisco binds a molecule of oxygen instead. When this happens, rubisco creates a toxic compound. The plant must go through a high-energy detox, called photorespiration, to neutralize the toxin.
The tobacco plants that South modified skip a few steps in this detox process, even though they reached the same end result. This saves the plant energy — energy it can divert to growth.
“To my knowledge, this is the biggest increase people have seen through this kind of genetic modification,” says Krishna Niyogi, a plant biologist at the University of California, Berkeley, who was not involved in this study. Niyogi is part of a larger international research collaboration that includes South: the $83 million Realizing Increased Photosynthetic Efficiency (RIPE) Project, funded largely by the Bill and Melinda Gates Foundation.
As impressive as it is, the 40 percent growth bump South achieved isn’t enough to feed the mouths of the future. We need to increase food production by 50 to 70 percent to meet mid-century food demands, according to that same 2013 study.
Luckily, photorespiration isn’t the only inefficient part of photosynthesis that can be improved with gene editing. RIPE Project researchers, including Niyogi, are working on genetic hacks that could be used in tandem with South’s photorespiration tweak to push plants’ efficiency to new heights.
For example, by genetically tweaking a process called photoprotection, Niyogi increased tobacco’s mass by 15 percent in a 2016 study. Direct sunlight can prove too strong for some crops, and they begin to give off heat — not unlike how humans give off heat while working in the sun.
A cloud passing overhead can disrupt the process: The plant continues to give off heat (read: waste energy) even though it’s no longer receiving enough light to fuel photosynthesis. Niyogi’s team sped up tobacco’s reaction to cloud cover and other disturbances in light, so that plants generate less heat when shielded from the sun.
Other RIPE Project researchers are trying to improve rubisco’s ability to grab carbon dioxide so it’s less likely to mistakenly grab oxygen and waste energy during photorespiration. To make this upgrade, researchers are inserting genes from organisms like bacteria and green algae into plants, which concentrate around rubisco and overwhelm any oxygen lurking in the area that would lead to wasted energy. But this type of research probably won’t be ready to test in crops for five to 15 years, says Alistair McCormick, a plant biologist at the University of Edinburgh in Scotland.
The RIPE Project aims to eventually provide efficiency-boosted crops to farmers in sub-Saharan Africa and Southeast Asia, places at high risk of food shortages, South says.
Many of the RIPE experiments started with tobacco plants because they are easy to genetically modify — the “lab mouse of the plant world,” South says. The next step for South’s team is to translate their success with tobacco to food crops. So far, they have successfully added the yield-boosting genes to potato plants. Now they are testing the modified potato plants to see if their new genes give them the same efficiency bump they gave tobacco.
South is optimistic that this technology and its efficiency effects can make the jump to crops like potatoes, soybeans, and black-eyed peas. Previous experiments that pumped carbon dioxide into the air around plants have shown reduced photorespiration in wheat and rice, increasing plant yield — essentially doing what green algae do for themselves, but without any genetic tricks. But airborne spraying of carbon dioxide isn’t practical for large-scale agriculture, so South and his colleagues are moving ahead with gene hacking.
Some experts, though, warn that translating South’s success to other crops won’t be easy. Food crops can be harder to genetically engineer than tobacco, says Raquel Carvalho, a plant geneticist at York University in England.
“They have very similar genes,” Carvalho says. “But it’s never going to be straightforward.”
While South is determined to translate his tobacco findings to food crops, Niyogi’s photoprotection research is further behind, though Niyogi thinks both efforts will ultimately succeed. “We haven’t yet gotten into doing field trials, but what worked in tobacco is likely to work in other crops,” Niyogi says.
Technical success, if it comes, won’t be the end of the story. The modifications will then have to win the support of governments and the public to improve crop yields around the world. That may prove to be a formidable hurdle: Half of U.S. adults think genetically modified foods are worse for their health. And while the National Academies of Sciences, Engineering and Medicine have declared that GMO foods are safe, less than 15 percent of U.S. residents agree, according to the Pew Research Center.
South hopes GMO skeptics will be more accepting of plants that are gene-edited for growth efficiency rather than for appearance or taste — or for pesticide and herbicide resistance, as with Monsanto’s controversial Roundup products.
Whether gene hacking to improve yields will affect plants’ susceptibility to pests is still unknown, says Jennifer Kuzma, co-director of the Genetic Engineering and Society Center at North Carolina State University. She says gene edits can have unintended effects on a plant, such as lowering natural resistance to pests, which may lead to more pesticide use.
While Kuzma is impressed with the project overall, she worries about the possible negative effects of farming the gene-edited crops. Her main concern is that the modified plants will breed with weeds, passing on their high-growth ability. This isn’t a huge risk though, she adds, since a whole complex of genes would have to be passed down to create the full yield-boosting effect.
Kuzma also worries that the gene edits could unintentionally alter the amount of toxins or nutrients in plants, though those affects could presumably be identified in early field tests of the modified crops. It’s also worth considering the ecosystem-wide effects of these crops, she adds, such as if they will require more water to grow.
Scientific concerns aside, Kuzma also harbors reservations about the technology for social reasons. “I’m not a fan of people that claim biotechnology can feed the world,” Kuzma says, “because if we could minimize food waste and get food to where it’s needed and eat less meat, we probably could feed people on a long-term basis.”
Even with public acceptance and roadblock-free testing, it could take a while to get efficiency-edited crops to market. Once South’s team successfully modifies the food crops, they will have to test the plants in farm fields in various climates. All in all, South estimates the process could take another 10-15 years and $150 million.
If the RIPE Project succeeds, ecosystems could benefit, too. Higher crop yields would mean that farmers could produce more food on less land, lessening the amount of deforestation needed for agriculture. Depending on how efficient scientists can make the plants, South says, it may be possible to decrease the amount of farmland used globally, freeing up more land for conservation.
But the key goal of the project is to feed people that would otherwise go hungry — especially those hardest hit by malnutrition and hunger, in regions like Southeast Asia and sub-Saharan Africa — even if it will take tens of millions of dollars to get there.