From fossil to fuel
Unearthing the millions-year-long process that fuels our world
Avery Orrall • January 11, 2024
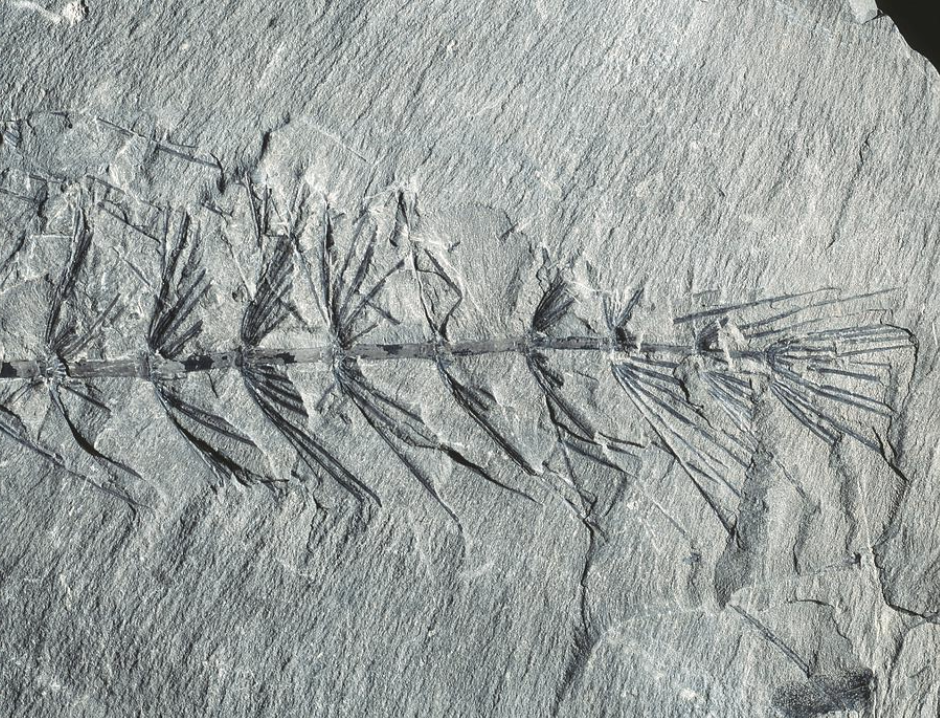
A fossil of the horsetail plant, which is often found in coal deposits and grew during the Carboniferous Period [CREDIT: Gilles Mermet | Fine Art America]
Have you ever wondered why we call oil, gas and coal fossil fuels?
It all started in the Carboniferous Period about 300 million years ago, appropriately named for the bloom of carbon-rich organisms during that time. When you hold your phone, fly in a plane or turn on the lights in your home, you can thank the plants and algae that lived and died all those years ago: the energy they created through photosynthesis is being used to power our world.
Where do these fossils come from?
The most common use of the term fossil, the durable impression a prehistoric organism leaves on a piece of rock, doesn’t really apply to fossil fuels. They are not made from rocks but rather from the decayed organism itself. People often believe dinosaur bones make fossil fuels, but that is generally incorrect too, says Eric Roberts, a geologist at the Colorado School of Mines. Although a dinosaur could become a fuel, there simply weren’t enough of them to create the fossil fuel reserves that we have today.
Instead, most fossil fuels were formed from much more abundant life forms: algae and plants. Because these organisms get their energy directly from the sun, they have a higher concentration of energy stored in their chemical bonds. A chemical bond is the force that holds atoms together in a molecule. Organisms higher up on the food chain, including plant-eating dinosaurs and finally meat-eating dinosaurs, store a lot less energy. This is because thermal energy is lost with each step of the food chain, as it takes energy to consume and digest other organisms.
How do fossil fuels form?
When algae die, they fall to the bottom of the ocean; terrestrial plants fall to the bottom of swamps. In both of those places, oxygen is sparse and organic matter quickly settles under layers of sediment. Over millions of years, these sediments then can harden into rock and subject the decayed organic matter underneath to extreme pressures at high temperatures (thanks to the heat of the earth’s core) — all without the presence of oxygen.
The process needs to be in low-oxygen environments so anaerobic decomposition can occur. In the presence of oxygen, which is a very reactive element, carbon dioxide would form as it does when we burn fossil fuels. The lack of oxygen deep underground allows the organisms to decompose while maintaining their carbon bonds. This also prevents aerobic bacteria from breaking the plants and algae down before they can turn into fuel.
Conditions have to be just right for this to occur. These low-oxygen environments can form only if the organic matter is buried relatively quickly before it has the opportunity to decompose in the presence of oxygen.
What is left from this decomposition is an insoluble organic material called kerogen, composed mostly of either lipids or lignans, depending on which organisms died to form the kerogen. Both types of fibrous compounds can store energy, but lipids are more common in algae and plankton while lignans are found most often in terrestrial plants.
Over time and under the extreme heat and pressure of being pushed deeper into the earth’s crust, the kerogen decomposes and chemical bonds begin to form between hydrogen and carbon atoms, which arrange themselves into long molecular chains called hydrocarbons.
What’s the difference between the three major fossil fuels?
Coal, petroleum and natural gas are all hydrocarbons derived from kerogen, but they often form in different ways and have different properties.
The first difference takes us back to lignans and lipids. Lignans, which come from land plants, are what Roberts calls “gas prone.” They form what is known as type III kerogen and then go on to form natural gas. Lipids, which come from algae and are “oil prone,” form the more energy-rich type I kerogen and eventually become oil or petroleum. A mixture of both lipids and lignans will form type II kerogen, which can produce both oil and gas.
The second difference comes from temperature. The kerogen cracks at around 50 degrees Celsius (122 degrees Fahrenheit), which means hydrocarbons start to form. The oil window is between 50 and 120 degrees Celsius, whereas the gas window is between 120 and 160 degrees, says Roberts. Anything in the 120-degree range may produce a “wet gas,” or what we might refer to as propane.
The least complex type of fossil fuel is natural gas, or methane. This compound can also be formed by methanogens, which are microscopic organisms that live in the intestines of animals and low-oxygen environments. This kind of methane is known as biogenic, whereas the kind formed from fossil plants is known as thermogenic. Methane is the least energy-rich fossil fuel, followed by propane and oil. Although most of it seeps up and escapes from the earth’s crust, some methane gets caught in salt domes and other rock formations.
Coal forms in an entirely different way: on swampy land with lots of vegetation and leaves, explains Steven Earle, geologist and author of the textbook Physical Geology. The early form of coal is peat, which is partially decayed organic matter that exists primarily in bogs. After the pressure and heat of burial over millions of years, the peat turns into coal. It is the most carbon-rich fossil fuel, so burning it creates the most carbon dioxide.
How does burning hydrocarbons produce energy and why is this a problem?
The process of burning is called combustion, and at the molecular level, it involves introducing oxygen to a substance. Setting fire to a fossil fuel causes oxygen to replace the hydrogen molecules bound to carbon. This rapid replacement releases the energy trapped in the chemical bonds, generating carbon dioxide, water, heat and light, says Earle.
Burning coal, oil and gas generates toxic air pollutants, including carbon monoxide and sulfur dioxide, and also releases massive amounts of carbon dioxide, a large greenhouse gas contributor to global climate change.
Roberts has focused his research on the future of energy. He studies carbon capture, or putting carbon dioxide from burning fossil fuels back into the earth for permanent storage to prevent global warming effects. He also does research on using hydrogen as an energy source.
“Make no mistake,” Roberts says, “I suppose we still need in the immediate future these resources, and we’ve got to keep understanding them. But I think the future is really quite an interesting and exciting time to be a geologist.” The better we can understand fossil fuels, the more confident we can be about finding a post-carbon energy future.
1 Comment
NICE ARTICLE. THANKS,. COME WEST AND WE WILL GIVE YOU A TOUR OF OUR JURASSIC AREA.